The Muon JRA – new technology for the future of muon research
By Stephen Cottrell, June 2013
Europe is fortunate in having two muon sources that are complementary and together offer researchers access to the full range of muon spectroscopic methods. The Muon Spin Resonance (μSR) technique is remarkably versatile, encompassing studies of magnetism, superconductivity and spin and charge transport, while providing a highly sensitive hydrogen analogue to probe semiconductors and proton conductors. The technique has an important role beyond condensed matter physics, and offers chemists a valuable method for investigating the fundamentals of reaction kinetics. It is also an excellent tool for the study of organic radical structure and dynamics in solids, liquids and gases. The beam structure of the SμS, located at PSI, makes it ideally suited for applications where high timing resolution is essential to follow fast muon precession or rapid spin depolarisation. In contrast, the pulsed nature of the ISIS beam, operated by the Science and Technology Facilities Council in the UK, allows low background time differential data to be captured at high data rates, while enabling the effect of beam synchronous excitations (such as Radio Frequency or laser radiation) to be easily investigated. The work of the JRA in Muons has stimulated development of a broad range of source technologies, while engaging the user community to research novel experimental techniques and analysis tools. Together, this work is now making a significant impact on European muon research.
Developing μSR in high magnetic fields
The development of new instruments for high magnetic field spectroscopy was essential to provide the user community with the tools necessary for future research. However, μSR in high magnetic fields is uniquely challenging. Both the implanted muons and detected decay positrons are charged and their trajectories are modified by the applied field. The detector systems need to be field insensitive yet capable of providing fast-timing. A significant aim of the JRA has been to develop the technologies required for high field μSR spectroscopy. The importance and success of this work is evidenced by recent grant awards to both facilities to develop high field instruments.
The ISIS instrument (fig 1) provides a 5T field parallel to the muon spin polarisation and offers a sample environment covering temperatures between 25mK and 1200K. The instrument is now fully integrated into the ISIS user programme and has already been used for a broad range of studies, with journal publications in frustrated magnetism, molecular nanomagnetism and molecular radicals.
In contrast, the spectrometer at the SμS (fig 2) is optimised for measurements where the 9.5T field is perpendicular to the spin polarisation. Test experiments have confirmed excellent performance through the measurement of full amplitude spin precession signals at approximately 1.29GHz in the 9.5T field. Fast timing at sample temperatures below 20mK has been achieved through the development of a novel optical readout for the dilution fridge. The instrument is currently being commissioned in preparation for user experiments.
Simulation codes and beam diagnostics to characterise muon and positron profiles
A comprehensive suite of simulation codes was developed to model both the profile of the muon beam through the instrument and the positron track to the detector. The program, musrSIM, based on the popular Geant4 toolkit, enables the instrument geometry, materials and field profile to be defined. It also allows us to study the response to muons and decay positrons. Input can be taken from beam simulation programs to accurately model the incident muon beam, while the response can be analysed and tested for various acquisition parameters using the associated application musrSimAna. The codes played a crucial role in the development of the detector arrays for both the ISIS and PSI instruments. As an example, figure 3 shows a study of the detector geometry used for the SμS spectrometer, and illustrates the need to consider positron spiralling when locating the positron counters.
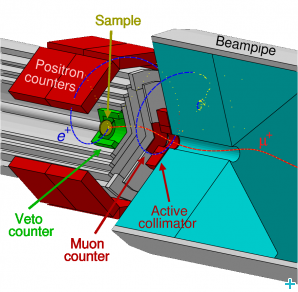
Fig 3. A study of the detector geometry and positron trajectories for the SμS high transverse field spectrometer
We checked our early simulation results during experimental work at the SμS, making use of a novel position-sensitive scintillating fibre detector developed within the JRA for profiling muon beams in high magnetic fields. Detailed beam profiles were obtained using a grid of 20 fibres covering an area of 10×10cm2, with a readout using avalanche microchannel photodiodes enabling a compact design and assuring insensitivity to magnetic fields. The development of the ISIS high field instrument required new technologies for direct imaging of muons, since we needed a detailed understanding of how the muon spot evolved in position and shape in the applied field at the sample position. We achieved this by developing a field-insensitive beam camera, using a high sensitivity cooled charged-coupled device (CCD) to image the light from a scintillating screen mounted on the sample stage of the cryostat. The device proved crucial to the commissioning of the spectrometer, enabling the muon spot to be monitored as automatic systems were developed to control and stabilise the beam position with the magnetic field.
Detector optimisation
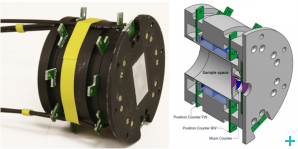
Fig 4. A prototype detector module based on avalanche photodiodes developed at the SμS (left) and schematic view
Significant work was carried out to optimise the detector arrays for high magnetic field measurements. For the ISIS instrument, although the scintillation detectors were, by necessity, positioned close to the sample, we successfully used extended light guides to move the field-sensitive photomultiplier tubes to a low field region. In contrast, the geometry and fast-timing requirements of the SμS spectrometer required a particularly compact detector array. We therefore had to develop a novel detector array based on Geiger-mode avalanche photodiodes (APD) for this instrument. We tested a prototype detector module (shown in fig 4) to 9.5T at the SμS and obtained excellent results. The measured time resolution was better than 80ps and, most importantly, was shown to be insensitive to magnetic fields.
Novel resonance techniques for new measurements
Novel NMR-style (nuclear magnetic resonance) pulsed radio frequency (RF) resonance techniques were explored as a means of obtaining new information from μSR experiments. Working in collaboration with a group at the University of East Anglia, two sequences were demonstrated during the project. Firstly, we investigated a double resonance method where both muon and nuclear spins are simultaneously irradiated to decouple the nuclear dipolar interaction. The technique is able to provide unique information about the muon site and dynamics in a system under study. Secondly, we explored a composite spin inversion sequence with the aim of improving the efficiency of RF spin rotation in RF μSR experiments. The pulsed nature of the ISIS beam was crucial to the success of both techniques – the RF excitation can be synchronised to the intense muon pulse, simplifying the setup of complex sequences and avoiding problems associated with RF heating. This work highlighted the need for good RF engineering of cavities to ensure reliable application of suitably intense RF pulses. To this end, we developed both an NMR system to enable off-line tests of RF cavities and a dedicated high power RF insert for the ISIS high field spectrometer.
μSR under Pressure!
Pressure is an important parameter in the investigation of the phase diagram of condensed matter systems and its study can often reveal new and sometimes exotic physical properties of materials. In the gas phase, compared to other resonance techniques, μSR provides a uniquely sensitive tool both for studies that can reveal spectroscopic information and enable a direct measurement of chemical reaction kinetics. An important activity supported by the JRA was the development of sample environment suitable for μSR measurements at high pressures in both the solid and gas phases. Work within this task was led by the group at Babes-Bolyai University and focussed on solving problems unique to μSR. For the solid sample pressure cells we investigated a number of designs with the aim of providing a reliable working pressure in excess of 2.5GPa even at cryogenic temperatures. The cells were configured to use the high energy muon beam available at PSI, and work was carried out to optimise the muon stopping range and maximise the sample signal. The provision of gas target pressure cells for the high field spectrometer was the focus of our work at ISIS, and we recently explored the spectroscopy of small gas molecules during commissioning experiments at the facility (fig 5). We continued this work by commissioning an integral RF cavity to enable RF techniques to be developed in the gas phase. The system is currently being used in collaboration with a group from University of British Columbia to investigate chemical reaction kinetics for simple abstraction reactions.
New software for data analysis: Simulation codes and Data Formats
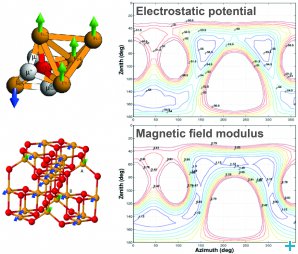
Fig 6. Visualisation of a structure and magnetic moment (left) and a search for muon sites by mapping electrostatic potentials and magnetic dipolar fields.
We have explored new software methods both to support the analysis of complex experiments and to help the community share data and software between facilities. Led by a team from the University of Parma, we developed code to calculate electrostatic potentials and magnetic dipolar fields within magnetic materials (fig 6). Experimental data can be compared to simulation results to help assign muon sites. Work continued to evaluate the potential for using Density Functional Theory (DFT) simulations to establish muon interstitial sites, and it was concluded that an ab initio strategy may provide a powerful solution provided the computational complexity can be solved.
The development of the NeXus data format provides a basis for storing muon data with comprehensive metadata to properly describe the experiment, a facility that will become increasingly important with the move to open access journals and data repositories. We carried out work to finalise a NeXus Instrument Definition suitable for both ISIS and PSI, while agreeing an essential subset of data and metadata for use by the worldwide muon community as a Common Exchange Format. This agreement should be beneficial to users as they move between facilities, and make it easier for the facilities and user groups to share software development effort.
Conclusions and future work
For the two European muon sources the work of this JRA has made a significant impact on the instrument suite available to their communities. Activities during both Framework Programme 6 (FP6) and the present JRA underpinned these projects through the development of technologies, such as field insensitive fast-timing detectors and simulation codes, essential for high field µSR. For the scientific community, work within the JRA has provided new equipment and methods for novel experiments that greatly extend the capability of μSR. Importantly, the JRA has given a focus to all these activities, providing resources for groups to work collaboratively to develop high quality long term solutions.
Looking to the future, during our second project under FP7 we are planning to transfer APD detector technology from PSI to ISIS, evaluating its suitability for use at a pulsed muon source. The on-going theme of high field μSR will continue, with work now focussing on the development of efficient algorithms for the analysis of high field experiments. We are also engaging in outreach through workshops, leaflets and websites, with the aim of bring the unique potential of high field μSR spectroscopy to the attention of the wider scientific community.